A NEW UNDERSTANDING OF HOW SEAM OPAL IS MADE IN NATURE By Gerard Faber
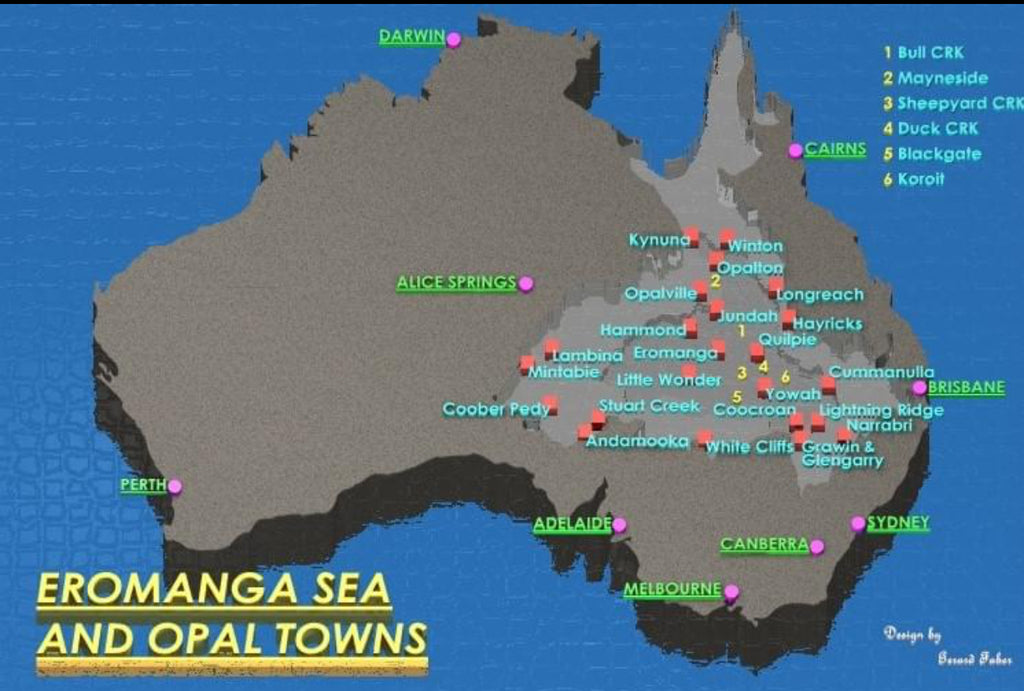
1. **Gondwana's Breakup**: The breakup of Gondwana led to the formation of the current continents, including Australia, which experienced significant climatic and environmental changes conducive to opal formation.
2. **Eromanga Sea**: The rise of the Eromanga Sea, which covered much of Australia during the Cretaceous period, provided the perfect conditions for diatoms to bloom and subsequently form diatomaceous earth.
3. **Role of Diatoms**: Diatoms, with their silica-based cell walls, played a crucial role in providing the silica necessary for opal formation. Upon their death, these diatoms settled to the sea floor, forming silica-rich sediments.
4. **Environmental Conditions**: The interaction of volcanic activity, glacial movements, and sedimentation processes over millions of years created the unique conditions necessary for the formation of opal-bearing layers. Volcanic ash provided additional trace elements essential for opal formation.
5. **Geological Processes**: The paper describes how various geological processes such as sedimentation, glaciation, and the impact of meteorites contributed to the formation of opal. These processes led to the creation of voids and cracks where silica-rich fluids could accumulate and eventually form opal.
6. **Chemical Processes**: The transformation of amorphous silica into opal involves complex chemical processes, including changes in pH levels and the involvement of various trace elements and minerals. The concept of zeta potential and the role of capillary forces in the aggregation of silica spheres are crucial for understanding opal formation.
7. **Modern-Day Opal Fields**: The paper also touches on the characteristics of modern-day opal fields in Australia, such as Coober Pedy, Lightning Ridge, and Andamooka, explaining the differences in geological structures and environmental conditions that affect opal quality and occurrence.
Gerard's research brings together geological history, chemical processes, and environmental factors to provide a comprehensive understanding of how seam and fossil opal is naturally formed, particularly in Australia.
See full paper below.
-------------------------------------------------------------------------------------------------------
"A NEW UNDERSTANDING OF HOW SEAM OPAL IS MADE IN NATURE".
A ground breaking scientific paper by Gerard Faber.
Have you ever wondered why Australia supplies 95 percent of the world’s opals. I am writing this paper to explain my understanding of how seam opal is made in its natural environment through the research that I have done on this matter. Gondwana was the great southern landmass that formed as a result of the division of a much larger supercontinent known as Pangea about 250 million years ago. This Gondwana supercontinent consisted of present-day landmasses: Africa, South America, India, Madagascar, and Australia. The last three continents to leave the Antarctic are South America, India, and Australia. Australia was the last to leave around 130 million years ago, at a rate of only a few mm per year.
The map of Gondwana depicted the Antarctic having ice on it but in reality, never did around the age of this map 200 million years ago.
The Antarctic was a warm humid place with forests, Cycads were the dominant plant. Australia has the same, forests mostly on the eastern side, and central Australia is Arid. For the 55my as the Australian content moves north, the climate has changed from tropical to two seasons, winter and summer. The sessions were extreme, Ice and snow in winter and hot in summer. There were even times when there was no summer and huge glaciers from the highlands on the eastern side of Australia worked their way onto the western side of the Eromanga Sea. We know this by the drop stones (rounded quartzite boulders) that are found in the mix of the kaolinite (Kopi). The boulders are picked up and moved to the edge of the glacier. The glacier would melt, and the boulders released. The concrete layer in the opal layers (consisting of finely rounded pebbles) is developed by the glaciers grinding its bottom along the ground picking up rocks and grinding them into fine small pebbles and sands compacting it.
The sea level was rising Because of all of the contents breaking up, creating easier access through the Gulf of Carpentaria to the interconnected basins that characterized much of Australia from down-warping and rifting. By its peak, between 115 and 110 million years ago all the inland basins were covered by a single, huge sea. Australia then consisted of 4 islands. The inland basins start from the top of Queensland and follow through to central South Australia and across to the top half of New South Wales.
The map shows the Eromanga Sea and the opal towns that exist.
The inland sea was perfect for Diatoms to bloom. Diatoms are 150-200 million years old and are Planktonic but only in the photic zone, meaning they float in a depth no more than 100 meters deep in the ocean’s currents. Diatoms are ubiquitous in both marine and freshwater environments. Diatoms are particular about the quality of water in which they live. For example, species have distinct ranges of pH levels where they will grow. Diatoms also have ranges and tolerances for other environmental variables, including nutrient concentration, suspended sediment, flow regime, and elevation. Diatoms are algae that live in houses made of glass. They are the only organisms on the planet with cell walls composed of transparent, opaline silica. Diatom cell walls are ornamented by intricate and striking patterns of silica. When an individual diatom dies, it will lose buoyancy, and the frustule will sink to the bottom of the sea floor. There are three ways diatoms die, eaten, in extremely cold climates, and in old age. The cold climate event is where the diatoms are floating in the ocean current. All the ocean’s currents flow freely past each other all over the world. Once the diatoms get trapped in this cold current, they will flow past the ice and instead of falling to the ocean floor they will be trapped in ice, that ice melts and then it is relisted. The old age is referred to as marine snow. It is where the Diatom dies of natural courses, also faecal matter and other inorganic dust all fall away to the ocean floor. In areas of dense diatom populations, this results in a sediment layer formed from a vast number of these discarded frustules. These sediment layers which are the diatoms are referred to as diatomaceous earth (kaolinite is the geological term / Kopi as the miners call it).
Just for an example of sedimentation of dead organisms is the White Cliffs of Dover the region of the English coastline facing the Strait of Dover and France. The cliff face, which reaches a height of 350 feet (110 m) and stretches for eight miles (13 km), owes its striking appearance to its composition of chalk accented by streaks of black flint, deposited during the Late Cretaceous. Around seventy million years ago this part of Britain was submerged by a shallow sea similar to Australia. The sea bottom was made of white mud formed from the fragments of coccoliths, which were the skeletons of tiny algae that floated in the surface waters of the sea. This mud was later to become the chalk from the deep weathering event.
The full name Coccolithophores are an important group of calcifying marine phytoplankton that during certain phases of their cycle of life are able to produce calcium carbonate plates called coccoliths that cover the cell in a structure called Coccosphere. Although coccolithophores are not silicified like Diatoms, some species exhibit a requirement for Si in the calcification process. Because diatoms rely on silica rather than calcium carbonate to build their shells, they were previously thought to benefit from ocean acidification. A chemical change in seawater triggered by the increasing uptake of CO2 makes calcification more difficult. This is the reason why the white cliffs of Dover have no opal. A major source of CO2 is from the “White and Smokers” which are chimneys formed from deposits of barium, calcium, and silicon, which are white. Underwater volcanoes at spreading ridges and convergent plate boundaries produce hot springs known as hydrothermal vents. They appear to shimmer because of differences in water temperatures or bubbles because of the presence of gases, like carbon dioxide.
On the ocean floor, the Diatomaceous earth is being fed on by mud-dwelling creatures. These creatures leave holes in the Diatomaceous earth the same as you see when you come across pipe opal in mining. The frustules of the Diatoms are now liquefied under pressure in the world seas and are called silica oozes (380 times greater than the atmospheric pressure at sea level which is 6.60 kg on average). The ooze is now free-flowing under gravity and invading the holes that the creatures have made. While the organic components aren’t long-lived, their silica exoskeletons can remain in sediment layers for millions of years and are responsible for the silica for the opal. Diatomaceous earth has the contents of major elements (SiO2, TiO2, Al2O3, Fe2O2, MnO, MgO, CaO, Na2O, K2O, O2O5, So3, Cl, BaO, and LOI). The contents of trace elements (V, Cr, Bi, Ni, Cu, Zn, Ga, Rb, Sr, Y, Zr, Nb, Mo, Sn, and Ta). These elements come from Whitsundays volcanoes; they flow through the volcanic vents in the Eromanga Sea. There are no Heavy Rare Earth elements in Diatomaceous earth. The Heavy Rare Earth elements in opal come from volcanic ash only.
Opal has the contents of trace elements (Zr, Nb, Na, Mg, Al, K, Ca, Sc, Ti, V, Cr, Mn, Fe, Co, Ni, Cu, Zn, As, Rb, Sr, Cd, Cs, Ba, Pb). The contents of Light Rare Earth elements (La, Ce, Pr, Nd, Sm, Eu). The contents of Heavy Rare Earth elements (Y, Gd, Tb, Dy, Ho, Er, Tm, Yb, Lu, Th, U).
The meteorite known as EET 83309 was found in Antarctica and has pieces of opal in it. The meteorite had entered the earth’s atmosphere at a great speed and extreme heat crashing into Antarctica where the ice was enriched in dead diatoms. Melting part of ice that was in close contact with the meteorite, depositing the amorphous Silica into the meteorite where it stayed untouched for thousands of years. Amorphous silica turned into opal. We discuss the mechanics of this later.
A false-color image of the narrow opal rim surrounding a bright metallic mineral inclusion in the Antarctic meteorite EET 83309; the circular holes in this image are spots where laser analyses have been performed. Image credit: Hilary Downes et al.
In an opal-bearing layer, there are important Items to successfully keep the moisture in. They are gypsum (only in seawater environment), concrete, Bentonite bed, and kaolinite. This is for Andamooka, Coober Pedy, white cliffs opal fields but may not necessarily be for Lightning Ridge. Lightning Ridge is made up of fresh water in a marine environment. The fresh water is from ice water melt but mainly from underground springs that flow freely into the Eromanga Sea under the water creating a barrier between salt and fresh water for millions of years. The Mintabie ground structure consists of sandstone to keep the moisture in. Mintabie is situated close to the border of the Eromanga Sea and the Sand dunes come to the edge via the arid lands of the western side of the Eromanga Sea. The wind blows the sand from the sand dunes straight on top of the inland sea where it falls away to the bottom just like the diatoms do to mixing together. Creating a coarser deposit which we call sandstone. The sandstone is formed over hundreds of years by lakes, rivers, and ocean floor deposits. These elements compress when they contact quartz and calcite. The pressure of these minerals coming together over time forms sandstone. Calcite is a carbonate mineral and the most stable polymorph of calcium carbonate. Combining (calcium oxide, CaO) with water to produce calcium hydroxide (Ca (OH)2), which is then mixed with carbon dioxide to form the calcium carbonate. Diatomaceous earth is made up of 19% calcium and there is an abundance of (carbon dioxide CO2) in seawater. The world’s oceans are a sink for the world's carbon dioxide. Coober Pedy is near Mintabie, and it also gets the fine grains of dust from the sand dunes causing the Kopi to be harder in places. Some of Andamooka’s fields also have harder Kopi than the majority of the rest of the fields. It all boils down to how much dust and fine sand are blown from the sand dunes onto the inland sea to how much harder the Kopi gets.
This picture is a fine example of Ice on the lake meets sand dunes. In the Eromanga Sea case not only ice but also glaciers.
110my ago the Whitsundays lay in a geologically active zone, where volcanic activity continued for 37 million years creating the Whitsundays islands. There were volcanic vents in the inland sea on the Whitsundays side of Australia. These vents spewed out all kinds of trace elements. As the diatoms float around the upper (0 – 100 meters) part of the water column, the diatoms collect trace elements. Diatoms are only 1 mm in size big enough to hold on to the trace elements. As the basins filled up with dead diatoms (Diatomaceous earth) over the 55my, the Whitsunday volcanos were erupting causing volcanic ash and underwater mudslides that would lay on top of the Diatomaceous earth. This creates a Bentonite bed which forms from the weathering of volcanic ash in seawater (fresh water for lighting ridge), which is in the colour of white, pale blue, and green beds. Volcanic ash has the contents of major elements (SiO2, TiO2, Al2O3, FeO, MnO, MgO, CaO, Na2O, K2O And P2O5). The contents of trace elements (S, V, Cr, Co, Ni, Cu, Zn, Pb, As, Mo, Rb, Sr, Ba, Zr, and Nb). The contents of Light Rare Earth elements (La, Ce, Pr, Nd, Sm, and Eu). The contents of Heavy Rare Earth elements (Y, Gd, Tb, Dy, Ho, Er, Tm, Yb, and Lu). Followed by a prolonged winter for thousands of years due to the volcanic gas and ash blocking out the sun’s rays in the atmosphere, this created a deep freeze to keep the glaciers more active in this area of Australia, and the concrete was formed from the glaciers grinding the ground underneath. The volcanic ash fell onto the glaciers and when the glaciers subsided the volcanic ash would fall away to the inland sea floor on top of the concrete. This is the start of an opal-bearing layer. This cycle keeps on happening over again until Whitsunday volcanic activity has ended. As the saltwater in the inland sea subsided and dried out, gypsum was formed in the ground around opal bearing layer. In Andamooka there are three opal-bearing layers (the false level, the main false level, and the main level). It was led to believe for some time that there were only 2 levels (false level and main false level which is known as main level until a man called (Merve Mundy) realized that there was one more opal bearing level underneath the second level, this is how the second level got its name (main false level). If this is the case it should be the same for the other opal fields too for the volcanic ash was spread out in a wide area.
The map shows the Eromanga Sea with its multi-layered depths and volcanoes.
65ym ago there was a great extinction happened. The asteroid hit the earth at Mexico's Yucatán Peninsula and the ground where it hit was high in sulfur deposits. The blast dispersed the sulfur into the earth’s atmosphere. The inland sea dried out. It also created the blast that put fractures through the diatomaceous earth where the Eromanga Sea was. This is where the extreme weathering started. The sulphuric acid rain leches through the cracks and crevices to liquefy the silica off the frustules of the Diatoms freeing the amorphous silica with the elements attached to it.
The metal cations from trace elements are staying in limbo due to the acid rain being only 2 Ph. The addition of amorphous silica from the Diatomaceous earth will cause the pH level to rise to 7 pH. The metal cations (positively charged metal ion) attach themselves to the silica spheres changing the silica spheres size. The stern layer and the electric double layer are divided into two parts separated by a plane (the Stern plane) located at about a hydrated ion radius from the surface. The centers of any adsorbed ions are located in the Stern layer, that is, between the surface of the silica sphere and the Stern plane. When the Amorphous silica reaches the position of depositing area the Ph level stays the same due to the volcanic ash Ph level of (8.5 to 9) This is where the silica sphere adopts the Light Rare Earth elements ions and Heavy Rare Earth element ions. This is the reason why opal is found mostly in the opal levels, but as the Amorphous silica parses through the level, and will be trapped in a void below inside the Kaolinite where it turns into opal there. As long as the Ph level is alkaline, and the metal Cations are attached to the silica spheres then the silica spheres can reach their zeta potential.
The liquid layer surrounding the silica sphere exists in two parts; an inner region (Stern layer) where the ions are strongly bound and an outer (diffuse) region where they are less firmly associated. Within the diffuse layer, there is a notional boundary inside which the ions and silica sphere form a stable entity. When a silica sphere moves (e.g. due to gravity), ions within the boundary move with it. Those ions beyond the boundary stay with the bulk dispersant. The potential at this boundary (surface of hydrodynamic shear) is the zeta potential. Zeta potential is the electrical potential at the slipping plane. This plane is the interface that separates the mobile fluid from the fluid that remains attached to the surface. The magnitude of the zeta potential gives an indication of the potential stability of the colloidal system. If all the silica sphere in suspension has a large negative zeta potential, then they will tend to repel each other and there will be no tendency for the particles to come together. However, if the particles have low zeta potential values, then there will be no force to prevent the particles from coming together and flocculating. The general dividing line between stable and unstable suspensions is generally taken at +30 or -30 mV (mV stands for Millivolts). Particles with zeta potentials more negative than -30 mV are normally considered stable. However, if the particles have a density greater than the dispersant, even though they are dispersed, they will eventually sediment forming a closely packed bed (i.e. potch). The initial zeta potentials increased with increasing pH, -20.3 mV at pH 4, -24.4 at pH 5, and − 26.3 mV at pH 6. This pH dependency is common with silica nanoparticles.
In certain circumstances, the particles in dispersion may adhere to one another and form aggregates of successively increasing size, which may settle out under the influence of gravity. An initially formed aggregate is called a floc and the process of its formation is flocculation. The floc may or may not sediment or phase separate. If the aggregate changes to a much denser form, it is said to undergo coagulation. An aggregate usually separates out either by sedimentation (if it is denser than the medium) or by creaming (if it is less dense than the medium). The terms flocculation and coagulation have often been used interchangeably. Usually, coagulation is irreversible whereas flocculation can be reversed by the process of deflocculation.
(a) Growth of the silica spheres starts at nucleation centers positioned randomly in the solution. Four of them are shown here. When the spheres reach a critical size (b). Electrostatic forces overwhelm entropic effects causing the spheres to line up in an ordered array. Water is uniformly distributed on the spheres as a wetting film (c). Growth continues until the solute is exhausted. A wetting film on the silica spheres Debye shells and a capillary neck between each two neighboring spheres (d). The distance between the centers of the spheres in optically active corresponds to the wavelength of visible light of a few hundred nm.
The superlattice constant of the ordered array depends on the number density of nucleation sites initially present. It is also possible that regular opal structures with lattice constants lying
outside the specific wavelength range needed to give electromagnetic activity in the visible spectrum do exist, you can see it in between colours where there is no colour, it looks like glass and no matter what angle you look at the opal it will always be crystal clear. The silica spheres would have to be 450nm and above to achieve this. That means that a silica sphere would be only 0.00045mm making it impossible to notice it with the naked eye unless it was built up upon itself with the same consistency in size. For the build-up of spheres of .5mm, it would have to be stacked 1250 times high.
Precise opal under an electron microscope in micrometers. Opal spheres are in nanometres. A nanometre is 1000 times smaller than a micrometer.
The conditions favorable for the formation of optically active are (1) a high surface charge density, (2) a long Debye length, and (3) an appropriate number density of nucleation centers.
Graphical representation of the water distribution using the model parameters obtained for the as-grown silica Colloidal crystal at room temperature ≈ 25 ºC. Water in the central sphere is not depicted to remark the wetting film. Where the overall capillary force also depends on the liquid phase connectivity, as it determines the bridge interfaces.
Demonstration of surface tension capillary action in the laboratory.
Capillary action occurs because water is sticky, thanks to the forces of cohesion (water molecules like to stay close together) and adhesion (water molecules are attracted and stick to other substances). It does not matter whether the groundwater is fresh water or salt water. Adhesion of water to the walls of a vessel will cause an upward force on the liquid at the edges and result in a meniscus that turns upward. The surface tension acts to hold the surface intact. Capillary action occurs when the adhesion to the walls is stronger than the cohesive forces between the liquid molecules. The height to which capillary action will take water in a uniform circular tube (picture below) is limited by surface tension and, of course, gravity.
The acid rain with the silica and the elements free-flowing under gravity through the cracks and fractures of diatomaceous earth. Falling deeper into the earth until it meets a void, a cavity, a boundary like the opal layer. The common elements come from the diatomaceous earth and the heavy/light rear earth elements come from volcanic ash at the opal level. When the silica, water, and elements stop moving it creates one completely loose silica cloud all mixed together. At this point, all the silica spheres in the silica cloud are repelling each other in a stable environment with a pH level of 8-9. The reason for being stable is through the Zeta potential. The silica spheres adopt the cations of the elements creating a stable charge of -30mV. A short time the silica cloud condenses. The majority of water on top of the silica cloud has a lesser number of elements compared to the silica cloud amount, causing the pH level of the liquid to drop to 6-7 pH. The silica spheres absorb the bulk of the elements inside the silica cloud. We now have a boundary called a liquid-solid boundary separating the liquid and the silica cloud. Once everything calmed down and there is no movement the silica spheres that are in contact with the liquid-solid boundary become immersed in the liquid side of the boundary. The amount is only a wafer, the wafer pills of continuously in an anti-clockwise direction. Because the liquid has a pH level of 6-7 and the energy of the silica spheres is about -26.3mV to -28.4mV it is causing the silica spheres to attract each other. It is known as flocculation. This is the most important part; it is the difference between forming opal and forming potch. Essentially Each silica sphere becomes an electrostatic charged and is attracted to the same size silica spheres. Creating one big jigsaw puzzle where they don’t interlock together but join together through capillary forces. Each silica spheres have a thin film of water around it. This adds the water necks to attach to each other and gives it the capillary forces needed to stay together. The spheres in the flakes now go through a change of size known as coagulation. All silica spheres to one flake are the same size. The silica spheres attached to the flakes are increasing size until the Debye length is exhausted or the flake becomes heavier under gravity and then it falls away. The longer the flakes are in the liquid boundary the larger the size and the higher up the colour spectrum. So now you can picture a whole heap of flaks of all different sizes according to their colour sizes on top of the liquid-solid boundary. The silica spheres show a blue colour at 359 nm, a green colour at 375 nm, and red colour at 502 nm. These flakes are getting bigger and bigger until their mass becomes too heavy for the liquid suspension to hold it in place and gravity takes over, it falls through the liquid-solid boundary and into the silica cloud. Remember that the silica cloud had a pH of 8-9 and the silica spheres are repelling from each other because it is in a stable solution. Well, this is in favor of the flakes. As the flakes descend through the silica cloud parsing each silica sphere, they give way to the flakes until they reach the bottom where they stay indefinitely. This is called sedimentation. This continues until the silica cloud is consumed or the elements have run out. Many of the time elements run out and we are left with just a silica cloud. The remaining silica cloud goes through sedimentation, then flocculation, and after that coagulation. This is the reason why there are more potch than opal out in the fields. The whole story happens all over again but next time it is not just to make opal or potch but to harden the two. Water is the natural hardener for opal or potch. With each addition of water, silica, and elements it hardens the others before it. As it is hardening the water film and water necks that are around the silica spheres are being used like a highway. All the elements are exhumed and repelled out through the highway to the outside of the opal and potch. All that is left are trace elements inside the gaps of the silica spheres.
Schematic diagram of the proposed growth mechanism for precious opal.
All the content has been independently researched by Gerard Faber and only facts are used, none of the content has been used or gathered from any other opal literature.
Leave a comment